Corrosion is a process where the metal can be degraded by electrochemical and/or chemical processes. Metals desire to be in their most thermodynamically stable state, which, in simplified terms, is the naturally occurring state of matter in its lowest energy state. Metals ordinarily exist naturally as oxides (e.g., iron oxide, aluminum oxide, zinc oxide, because oxides represent their lowest energy state). As corrosion is normally accelerated by the presence of water, oxygen and salts (particularly of strong acids), the function of a protective coating system is to maximize protection of metal substrate from these forces.
Oxidation occurs at the anode (positive electrode), and reduction occurs at the cathode (negative electrode). Corrosion is normally accelerated by the presence of water, oxygen and salts (particularly salts of strong acids).
Corrosion process in the case of steel (Figures 1 and 2) below.
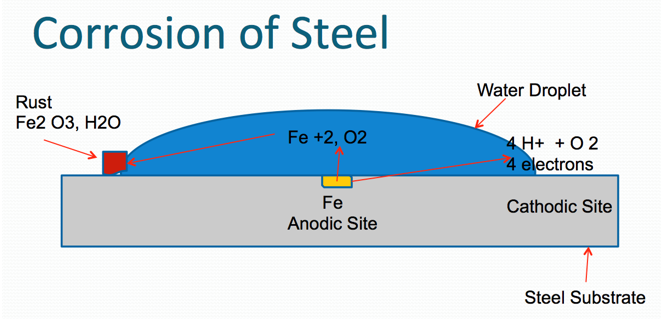
This article will consider the influence of the following formulating factors on the corrosion resistance of a coating.
- Type of metal
- Pigment level and selection
- Corrosion inhibitive pigments
- Service environment considerations and new innovations
1. Type of metal
According to the EMF series, aluminum and zinc are more active than iron and oxidize more quickly when exposed to oxygen and water. However, the oxides of uncoated zinc and aluminum form a tightly bound layer to the metal surface that decreases the rate of corrosion of the underlying metal. Whereas when carbon steel rusts, the corrosion product is ferric oxide that is loosely attached to the surface that is prone to more rapidly oxidize.
In the EMF series (figure 3 below), Zn is more active than Fe. When a zinc-rich primer is applied over steel, or in the case of galvanized steel, zinc will oxidize preferentially to steel and thus prevent the underlying steel from oxidizing. In this scenario, Zn is anodic (more readily oxidized) to steel and therefore protects steel from oxidation. Thus, steel is protected from corrosion by cathodic inhibition, as well as by the barrier that the zinc-rich primer provides.
2. Pigment considerations
The PVC (pigment volume concentration) of a system is defined as the volume percentage of solid particles in the system after film formation, when all volatile ingredients such as solvents and water have evaporated. The level and type of pigment used in a primer not only affects initial coating adhesion, but also its longevity while in service. Most primers are formulated at or slightly below Critical Pigment Volume Concentration (CPVC) to maximize topcoat adhesion (rougher primer surface and higher free energy) as well as many other coating properties (Figure 4).
PVC and the relationship between PVC to Critical Volume Concentration is a critical consideration and governs not only mechanical properties, but also influences moisture and oxygen permeation through the coating film to the metal substrate. Depending on the application and the desired mechanical properties (e.g., substrate adhesion, flexibility, topcoat adhesion, sandability), corrosion-resistant primers are formulated at various PVC’s and contain a variety of inert pigments.
The use of more polar pigments may provide ease of wetting during the pigment dispersion process, but may degrade long-term adhesion as they are more susceptible to moisture migration and disbondment at the coating-substrate interface. Plate-like pigments and pigments that have very low or no water-soluble components also enhance longevity.
Pigment particle size, shape and structure can influence moisture and oxygen permeation and ultimately corrosion resistance. Pigments with platelet-shaped particles can reduce permeability, especially if they are aligned parallel to the coating surface. Mica, micaceous iron oxide and metal flakes are a few examples of such pigments. These platy pigments provide a more torturous path water, soluble salts and oxygen to reach the metal surface. Other pigments that contribute to corrosion resistance include Platy aluminum silicate and Wollastonite (calcium silicate).
3. Corrosion inhibitive pigments
As detailed above, PVC and selection of select inert pigments influence barrier properties of a coating and enhance corrosion resistance. Corrosion inhibitive pigments impact the rate of corrosion by two main mechanisms, cathodic and anodic inhibition. Cathodic inhibition inhibits corrosion by impeding the flow of electrons at the cathode, whereas anodic inhibition inhibits corrosion by impeding the flow of electrons at the anode.
When choosing a corrosion inhibitive pigment, several factors must be considered. Environmental factors that influence the rate of corrosion include moisture, pH of the moisture, wet and dry cycles, soluble salts, temperature and time. With these issues in mind, the evaluation criteria and test methods must be carefully contemplated before selecting corrosion inhibitive pigments. Corrosion inhibitive or passivating pigments promote the formation of a barrier layer over anodic areas, thus passivating the surface. To be effective, these pigments have a minimum solubility. If the solubility is too high, the pigment will leach out of the coating too rapidly, reducing the time that the pigment is available to inhibit corrosion. If the coating film is more open (e.g., air dry latex), water permeation is higher, and thus the corrosion inhibitive pigment will be depleted more rapidly. To function properly, the coating must permit the diffusion of some water to dissolve the pigment. Accordingly, blister formation may result under humid conditions as the pigment dissolves. Higher Tg (glass transition temperature) and higher cross-link density binders are known to improve blister resistance.
The vast majority of corrosion inhibitive pigments are comprised of the combination of metal ions (cations) derived from zinc, strontium, chromium, lead, molybdenum, aluminum, calcium or barium and anions, such as those derived from phosphorous (orthophosphoric and polyphosphoric acids), chromic acid and boric acid. Although chromate and lead, containing passivating pigments, are very effective in inhibiting corrosion, their use is very limited due to a variety of environmental and toxicological regulations.
Another prime consideration in the selection of a corrosion inhibitive pigment is the pH. For example, a pigment with a high pH may have a deleterious effect on the cure of acid-catalyzed systems. Conversely, a pigment with a low pH may adversely affect the stability of waterborne systems.
4. Service environment, considerations and new innovations
The relative corrosion resistance of coatings can vary dramatically depending on the test method and exposure conditions. Common test methods include salt spray (95% humidity/5% salt and always moist), acidic salt spray, prohesion cyclic corrosion (wet and dry cycle with 0.04% ammonium sulfate and 0.05% salt), electrochemical impedance spectroscopy and salt soak. Most experts agree that accelerated tests are not always a good indication of how the coated metal will perform in the real world.
Additional considerations are the metal type (e.g., steel, aluminum, galvanized), pretreatment and cleanliness of the surface. If the metal surface is not properly cleaned and prepared, the coating will lack adequate adhesion and premature failure will result.
Furthermore, the type of coating in which the pigments will be used affects the selection of appropriate corrosion inhibitive pigments. Considerations include whether the coating is solvent-borne, waterborne, powder, air dry or baked, and if the film will be cross-linked or thermoplastic.
Other formulating factors that have a profound influence on substrate corrosion include the degree of hydrophobicity of the coating. Surface and volume hydrophobicity can be increased by the use of surface modifiers of specially designed/structured pigments as well as the addition of hydrophobic additives that minimize moisture permeation of the coating and thus decrease the rate of corrosion.
It is our experience that a coating with a high contact angle and volume hydrophobicity will also provide excellent retention of adhesion after accelerated testing such as salt spray or condensing humidity.
Two-component polyester urethane with a 155-degree contact angle and excellent volume hydrophobicity formulated in the laboratory of Chemical Dynamics, LLC
A sampling of suppliers of Corrosion Inhibitive Pigments include: